News Story
Powerful X-ray Beams Unlock Secrets of Nanoscale Crystal Formation
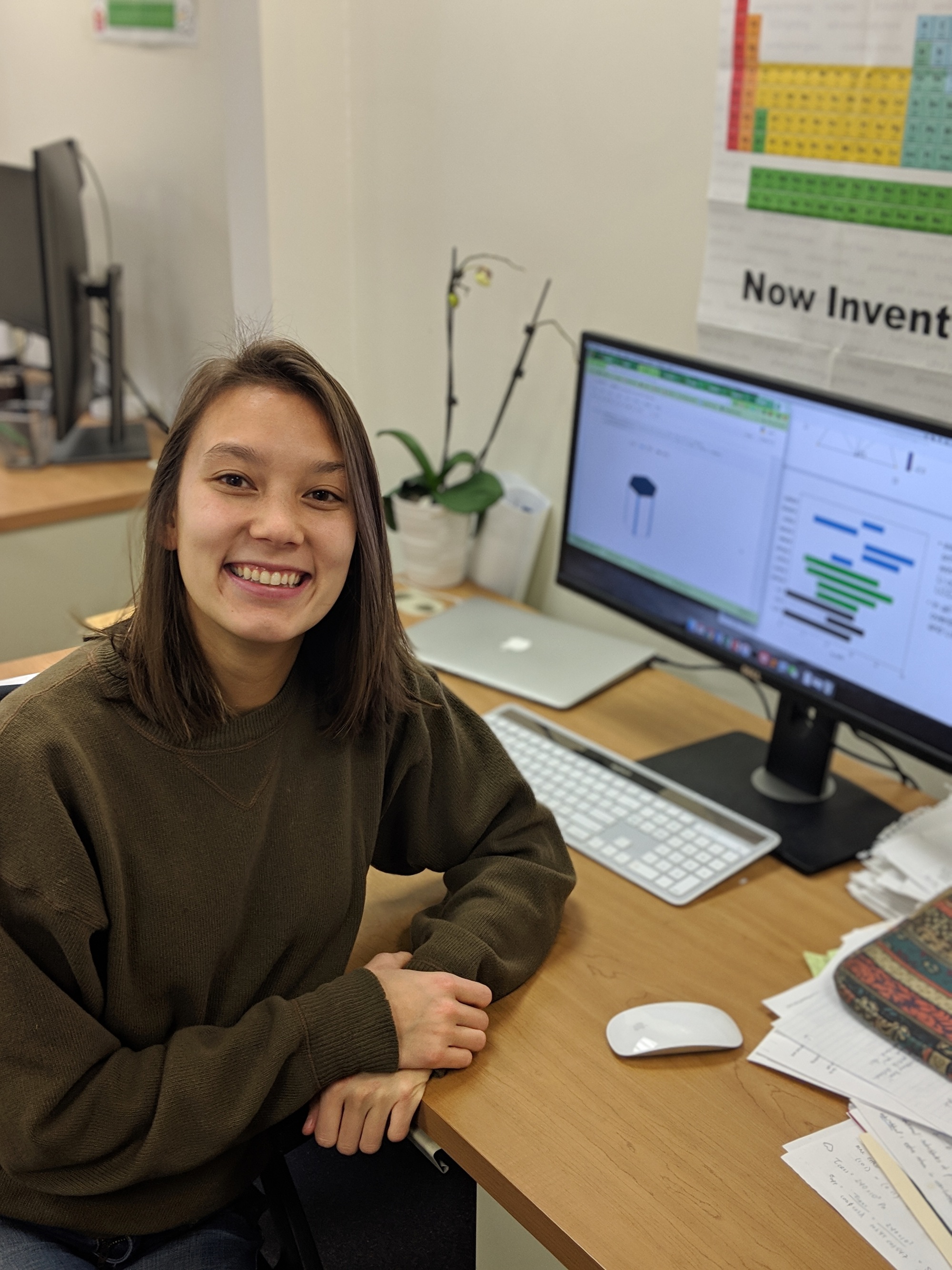
1) Adelaide Nolan, MSE grad student and second author, conducting her current research in the Mo Research Group lab.
High-energy X-ray beams and a clever experimental setup allowed researchers to watch a high-pressure, high-temperature chemical reaction to determine for the first time what controls formation of two different nanoscale crystalline structures in the metal cobalt. The technique allowed continuous study of cobalt nanoparticles as they grew from clusters of tens of atoms, to crystals as large as five nanometers.
The research provides the proof-of-principle for a new technique to study crystal formation in real-time, with potential applications for other materials, including alloys and oxides. Data from the study produced “nanometric phase diagrams” showing the conditions that control the structure of cobalt nanocrystals as they form.
This research, reported November 13, 2018, in the Journal of the American Chemical Society, was a collaborative study conducted by the University of Maryland’s (UMD) Department of Materials Science and Engineering (MSE); the Georgia Institute of Technology’s Department of Mechanical Engineering; Brookhaven National Laboratory, and Argonne National Laboratory. Xuetian Ma – a graduate research assistant at Georgia Tech – served as the research paper’s first author. UMD MSE Assistant Professor Yifei Mo and his colleagues, Adelaide Nolan and Shuo Zhang, served as co-authors.
The group demonstrated how altering the surface energy of cobalt nanoparticles through pH could entirely change the morphology of the particle.
In bulk cobalt, crystal formation favors the hexagonal close-packed (HCP) structure because it minimizes energy to create a stable structure. At the nanoscale, however, cobalt also forms the face-centered cubic (FCC) phase, which has a higher energy. That can be stable because the high surface energy of small nanoclusters affects the total crystalline energy.
“When the clusters are small, we have more tuning effects, which is controlled by the surface energy of the OH minus group or other ligands,” said Hailong Chen, assistant professor of mechanical engineering at Georgia Tech, and PI on the study. “We can tune the concentration of the OH minus group in the solution so we can tune the surface energy and therefore the overall energy of the cluster.”
The team examined the polymorphic structures using theoretical, experimental and computational modeling techniques. Experimentally, they reduced cobalt hydroxide in a solution of ethylene glycol, using potassium hydroxide to vary the pH of the solution. The reaction takes place under high pressure – about 1,800 PSI – and at more than 200 degrees Celsius. The team used a heavy steel containment vessel that allows them to analyze only the reaction results. To follow how the reaction took place, they needed to observe it in real time, which required development of a containment vessel small enough to allow for X-ray transmission while handling the high pressure and high temperature at the same time.
The result was a reaction vessel made of a high-strength quartz tube about a millimeter in diameter and about two inches long. After the cobalt hydroxide solution was added, the tube was spun to both facilitate the chemical reaction and average the X-ray signal. A small heater applied the necessary thermal and a thermocouple measured the temperature.
Data obtained by varying the pH of the reaction produced a nanometric phase diagram showing where different combinations produced the two structures.
The Mo Group used density functional theory (DFT) calculations to evaluate the surface energy of several low-index facets of FCC and HCP cobalt, with OH- as a surface adsorbent. Nolan found that the surface energy of each structure varied depending on the concentration of OH-, which is equivalent to the pH.
“By comparing the total energy, or the combined surface and bulk energy, of each phase, we constructed a phase diagram that indicated the predicted phase at a given particle size and pH,” she said. “The phase diagram agreed well with experimental characterization, and matched with nucleation theory that indicates how surface energy becomes increasingly important at the nanoscale.”
This methodology could have implications for directing the synthesis of nanomaterials, where the dominant phase is much more dependent on the surface energy than in the bulk.
“This work demonstrates the possibility to construct nanoscale phase diagrams for many other materials,” said Professor Mo, “where the particle size and chemical environment could be used to dictate synthesis.”
As a next step, the researchers plan to study alloys, to further improve the theoretical model and experimental approach.
For additional information:
Ma, X., Nolan, A.M., Zhang, S., Bai, J., Xu, W., Wu, L., Mo, Y., Chen, H. (2018) “Guiding Synthesis of Polymorphs of Materials Using Nanometric Phase Diagrams,” Journal of the American Chemical Society, DOI: 10.1021/jacs.8b11029.
*Thumbnail photo: GA Tech GRA, Xuetian Ma, hodling a reaction vessel for studying nanocrystal formation. (Credit: Allison Carter for Georgia Tech)
Reprinted with permission.
Published January 9, 2019