News Story
Spinning Engineered Silk Into Tiny Designs—With A Microscope
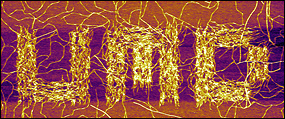
BioE senior Sara Johnson and her colleagues in Professor Joonil Seog's research group used an atomic force microscope to mechanically guide SELP nanofibers, forming the letters "UMD."
Johnson, a member of Department of Materials Science and Engineering (MSE) assistant professor Joonil Seog's research group, is part of a team investigating how an atomic force microscope (AFM) can be used to grow and weave threads of silk-elastin-like peptide polymers (SELPs) into specific patterns or shapes—such as the letters "UMD" at only one thousandth of a millimeter wide. She is the first author of a recent paper about the work published in Chemical Communications, one of the premiere journals in its field.
SELP is a polymer made out of alternating segments of genetically engineered spider silk and elastin, the protein responsible for giving skin and blood vessels their flexibility. SELP is studied because of its similarity to amyloid fibers, the suspected culprit behind certain neurological disorders such as Alzheimer's disease, and because of its potential applications in tissue engineering.
AFM employs a cantilever with a pointed tip—something like a very tiny diving board or the tone arm of a record player—to "see and feel." While the cantilever either taps or skims the surface of a sample, a laser tracks the location of its tip. As the tip's location changes, it deflects the laser, which is in turn detected by a photodiode. Ultimately, data collected about motion of the tip are translated into a three-dimensional surface map. The cantilever can also be used to measure mechanical properties at the single molecule level by pulling on a sample and characterizing force profiles.
Left alone, silk-elastin peptide polymer will naturally self-assemble into a hydrogel in a solution at body temperature. The Seog Group was the first to discover that the AFM's tapping action not only stimulated the formation of SELP fibers, but could also be used to direct them into specific patterns or shapes perpendicular to the microscope's scanning direction. While it has not yet been firmly established why interaction with the AFM's cantilever has this effect on SELP—that's the subject of the group's next study—Johnson's recent paper outlines the scanning conditions that optimize the fibers' growth, the relationship between scanning speed and growth rate, and what is known about the growth mechanism to date.
The three things that affect SELP assembly and direction, Johnson explains, are the AFM's scanning speed, cantilever tip pressure, and how many times the tip is scanned across the width of the sample, a variable known as line density. Johnson and her colleagues found that while using the AFM in tapping mode, lower scanning speeds, higher pressure, and increased line density (particularly once each line equals the width of a SELP molecule) resulted in the fastest and most directed growth. They also discovered that a SELP molecule on a mica subsrate must be tapped multiple times to stimulate nucleation, the process that causes the nanofibers grow. The creation of the miniature letters "UMD," says Johnson, was "the conglomeration of the best conditions [the team] found."
Going forward, the Seog Group hopes to understand the nucleation mechanism that occurs at the cantilever's tip, both why it happens and why the SELP fibers grow in the specific direction they do. Once that knowledge has been perfected, Johnson adds, she and her colleagues can begin to explore practical biomedical applications of structures made out of SELP.
Johnson says working on the project over the past two years and publishing her first paper have been fulfilling, and have inspired her to continue her education. "Coming into the undergraduate program, I didn't have any intent of going on to graduate school," she says. "I just wanted to get out there and make medical devices. Working with grad students and postdocs really allowed me to see that I could obtain [their] level of knowledge. Learning more about the research process can be very challenging, but you also have those exciting moments where you discover something new…and it's pretty rewarding!"
In addition to Seog, Johnson's co-authors on the work are Young Koan Ko (MSE), Nitinun Varongchayakul (MSE), Sunhee Lee (Korea Advanced Institute of Science and Technology), Joseph Cappello (University of Utah), Hamidreza Ghandehari (University of Utah), Sang Bok Lee (Department of Chemistry and Biochemistry), and Santiago D. Solares (Department of Mechanical Engineering). The work is part of an ongoing collaboration between the Seog and Solares research groups.
For More Information:
See: "Directed patterning of the self-assembled silk-elastin-like nanofibers using a nanomechanical stimulus." Sara Johnson, Young Koan Ko, Nitinun Varongchayakul, Sunhee Lee, Joseph Cappello, Hamidreza Ghandehari, Sang Bok Lee, Santiago D. Solares and Joonil Seog. Chem. Commun., 2012, 48, 10654-10656. Abstract »
Visit Professor Joonil Seog's Molecular Mechanics and Self-Assembly Laboratory web site »
Visit Professor Solares' homepage »
Published December 5, 2012